Radio Frequencies: 7 Most Asked Questions for the CWNA
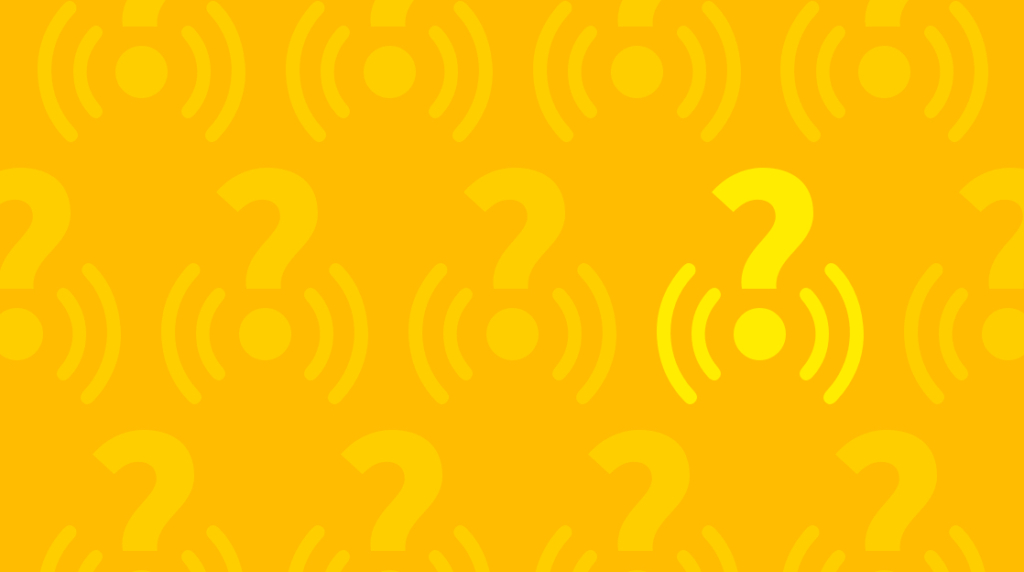
Try and imagine a world without radio. Maybe you shrug and think, “Well, I use Spotify, so I don’t really need radio.” But commercial radio is only one and increasingly tiny use of radio. A world without radio is a world without cell phones, Wi-Fi, microwaves, wide area networks, bluetooth and pretty much everything else that places us in the modern world. Oh, and we wouldn’t be able to see anything either.
The terminology, science and math behind radio frequency, sometimes called RF Theory, can be daunting, but we want it to make sense. We made a list of the most common questions and misunderstandings of radio frequency and brought it together here for you. Maybe you’re studying for a certification exam about RF theory. Or maybe you’re just trying to understand why, exactly, your Wi-Fi router uses so many different numbers and letters to explain what devices it can handle. Either way, read on for our intro crash course to RF theory.
What are Radio Frequencies?
The quickest definition of a radio wave is that it’s an electromagnetic wave that falls within a certain range of frequencies — specifically 3kHz to 300GHz specifically. Now, there are many different electromagnetic waves, some, like gamma rays and x-rays, carry a lot of energy. Others make visible light and microwaves possible. Radio frequencies are any of the electromagnetic wave frequencies that lie in the range extending from below 3 kilohertz to about 300 gigahertz, including frequencies used for communications signals.
Imagine dropping a pebble in a still pond; the wave ripples outward. Now imagine that same wave effect in three dimensions. That’s essentially what electromagnetic waves are doing. electromagnetic waves (EM waves) move energy through space in the form of a wave (or a waveform. An EM wave has a top and a bottom. As it travels it moves upward, moves downward, and then goes back up to its top.
EM waves are measured according to how often they repeat their up and down motion. That’s why it’s termed frequency. The word to describe how often a wave makes it back to the top of its pattern is oscillation, which is measured per second. One hertz (abbreviated Hz) is one wave per second.
That might sound overly technical, but it’s about as simple an explanation as you can get for how electromagnetic waves and radio frequencies work. The thing to remember is the difference between a gamma ray shooting through space, visible light bouncing on our eyeballs, and radio waves carrying Wi-Fi data to our phones is simply how much energy is in them — and that makes their frequencies change. If you measured all the possible electromagnetic waves according to how fast they go up and down, radio waves are way low on the list.
Radio frequencies range from 300GHz at the high-powered end to 3kHz on the low end. Now, they might be weak relative to other EM waves, but we use them all the time. They’re very common and they’re used in mobile phones, computers, and televisions as well as in many forms of broadcasting and communication.
What are the Physical Attributes of Wireless Communication?
It’s well and good to understand what an electromagnetic wave is and where radio frequencies fall in that, but something doesn’t just become a radio wave. It has to get there somehow. To grasp that, an understanding of the physical, non-wireless components can help.
Wireless communication depends on three physical things: a transmitter, a channel and a receiver. What they are, what constitutes them, and how they do their job change from system to system, but the physical attributes of wireless communication at their simplest are:
A transmitter that converts an electric signal into a form suitable for transmission
A channel which is the pathway between the transmitter and receiver
A receiver which is responsible for picking up the signal and reversing the process that restores the transmitted signal.
There are many different methods for converting signals and for forming a pathway, but no communication can be converted into a radio wave and used to communicate without these three physical components.
What are Decibel, mW, and Decibel-milliwatts?
You might be surprised (and dismayed) to learn that math is important in wireless communication. Let’s revisit the idea an EM wave is like a dropped pebble. The waves that ripple out from a small pebble dropped in the center of a lake won’t reach the shore. To get your wave to reach the shore, you’d either need a bigger pebble, or a complicated arrangement of pebbles that drop along the way to the shore, re-forming the ripples when they get weak. A similar thing happens with EM waves: they weaken over distance and time. Predicting and doing something about that loss requires math.
The basic unit of power is a watt, which measures the rate of energy transfer in a circuit. Our RF math will measure power in milliwatts (mW).
A decibel is a unit of comparison that measures the difference between two power levels.
That’s important because if a signal lost power while it was in transit, we’d want to quantify the change in power. For example, we could measure the rate at which we lose power. We might measure that we lose 100db of power for every 1000 feet of cable. RF math depends on decibel-milliwatts (dBm) per 1mW to measure loss.
Explaining more than the abstract idea of RF math is a cottage industry unto itself. But one of the most important rules in RF math is the rule of 10s and 3s to calculate the relationship between mW and dBm loss. The rule is:
For every loss or gain of 10 dBm, divide or multiply the power in mW by 10
For every loss or gain of 3 dBm, divide or multiply the power in mW by 2.
How Do You Measure Signals?
From here we progress into some of the more technical aspects of RF theory and troubleshooting wireless networks. One key part of maintaining a wireless network is identifying the signal strength. There are two technical measurements we’ll look at: EIRP and RSSI, both of which represent different parts of signal strength.
Equivalent Isotropic Radiated Power, or EIRP, indicates the strength of the signal from its radiation point. In other words, it’s the amount of signal leaving the antenna. EIRP tends to be expressed as dBi (power density with respect to an isotropic antenna) or dBd (power density with respect to a dipole antenna). The formula for measuring EIRP is [Transmit Power] – [Cable Loss] + [Antenna Gain].
Received Signal Strength Indicator, or RSSI, determines the total energy of a signal on the receiving end. RSSI is measured in decibels from 0 to -120. The closer to 0, the stronger the signal. The actual RSSI + the antenna gain will equal the Displayed RSSI.
For example, if a client card is a 2dbi antenna, and the actual RSSI is being seen as -55dBm, we plug those numbers into the formula to find our displayed RSSI. -55dBm + 2dbm = -53dBm.
How Do You Identify Noise?
Noise is any unwanted radio frequency energy being transmitted or received along with the desired signal. Noise exists in all RF systems to some degree, but how much and how strong it is can affect the system’s quality and performance.
The performance of a receiver or transmitter can be quantified with a ratio called Noise Figure, or NF. It’s calculated by comparing the signal-to-noise power ratio at the input to the signal-to-noise power ratio at the output.
Noise is usually categorized by how it’s generated:
Phase noise manifests in the form of sidebands that spread out from both sides of the signal.
Thermal noise occurs as charge carriers in a conductor increase the temperature.
Flicker noise is sometimes also known as pink noise or 1/f noise. It is any noise with a power spectral density that is the proportional inverse of the signal's frequency.
Avalanche noises can happen when a junction diode operates near the point of an avalanche breakdown.
Shot noise can be caused by fluctuations in the electrical current.
Burst noise sounds like a burst or pop. It occurs in some circuits where a semiconductor experiences a sudden impulse.
What are Wireless Bands and Channels?
Bands and channels can be difficult to understand conceptually, but they’re important for understanding wireless communications. First, it’s important to remember that a frequency is a physical thing. It’s the precise rate of up-and-down motion of the wave. Bands and channels, however, are human inventions to help explain how we use that behavior.
A band is actually a range of frequencies. Technological limitations mean that when different devices tune to the same frequency, they might be off a little bit. The band is a predefined range of frequencies that are considered grouped together. For instance, the band of EM energy we recognize as visible light typically exists between 380 and 700 nanometers. In other words, the light band is 380-700nm, but where red ends and orange starts needs a further subdivision.
A channel is, in essence, a specified, repeating subdivision of a band. When a band is channelized, it means the divisions between each broadcast frequency has a specific size. Citizen Band or CB radio, for instance, has 10KHz channels.
There are multiple bands in the radio spectrum that are used for Wi-Fi. Within these bands are many channels. A group called IEEE is responsible for laying out the definitions of how these frequencies, bands and channels are best implemented. IEEE defined many bands and frequencies, and the set of regulations that apply to wireless communications are designated “802.11”.
802.11 first defined the 2.4GHz band for use in wireless. The 2.4GHz has 14 channels, of which 11 are used in North America. This band’s 14 channels are spaced 5MHzapart.
802.11 specifies that wireless needs about 20 megahertz in channel width to operate. This can lead to odd technical challenges, since channels are spaced 5MHz apart and there can be overlap and interference from the adjacent channels. For this reason, you will find that equipment is typically deployed on channels 1, 6, and 11, since they’re the only channels that have no overlap.
The 5GHz band's channels are 20MHz wide, but do not overlap. The 5GHz band is further divided into UNII-1, UNII-2, UNII2e and UNII-3 "Unlicensed National Information Infrastructure" bands. It's worth noting that 5GHz has a shorter range, because it has a higher frequency than 2.4GHz band.
To put it concisely, 2.4GHz can travel longer distances but transmits at a slower speed. It is used in cordless phones, microwaves, Bluetooth, and wireless cameras. The 5GHz band provides faster data transfer rates, and is less crowded, with more channels, but it has a shorter range.
What are the Differences in the 802.11 Standards?
Like so much else in RF, exactly what the 802.11 standards are can be confusing. Like we mentioned, 802.11 refers to the regulations and standards for LANS and WANS. But technology keeps evolving, so the 802.11 standard does too. As capabilities have been added, the standards that unify them have been recognized as amendments to the standard. But at some point, it was realized that all the letters didn’t make sense to the broad public, so they also added a simple numbering convention so people could recognize them a bit better.
Version | Standard | WiFi Alliancenaming |
Original | EEE 802.11 | |
2 | IEEE 802.11a | Wi-Fi 1 |
3 | IEEE 802.11b | Wi-Fi 2 |
4 | IEEE 802.11g | Wi-Fi 3 |
5 | IEEE 802.11n | Wi-Fi 4 |
6 | IEEE 802.11ac | Wi-Fi 5 |
7 | IEEE 802.11ad | |
8 | IEEE 802.11ah | |
Latest | IEEE 802.11ax | Wi-Fi 6 |
802.11a/b. The "a" version is 5GHz with data rates of 54Mbps. The "b" version reverted to 2.4GHz and boosted data rates to 11Mbps.
802.11g continued to use the 2.4GHz frequency and had an increase to 54Mbps.
802.11n used both 2.4GHz and 5GHz. This offered both speed and distance as well as backwards compatibility with 802.11a/b.
802.11ac made a big jump to 400Mbps utilizing the 5GHz frequency.
802.11ad, compared to its predecessors, came to the table with something new. While the previous versions used either 2.4GHz or a 5GHz frequency, the 802.11ad used a new frequency: 60GHz.
802.11ah. Wireless range has to take into consideration distance as well as physical obstructions. The 802.11ah, operating in the 900MHz range can penetrate walls and other surfaces more easily, and with less power but at the sacrifice of speed.
802.11ax or Wi-Fi 6 is the most recent and most exciting wireless standard and it packs in improvements:
Multi-user MIMO which enables communication with multiple devices at the same time, available in both downlink and uplink directions.
Orthogonal frequency-division multiple access or OFDMA, which allows simultaneous low-data-rate transmission from several users, collision avoidance and better high-use performance by enabling more clients to connect to a single access point simultaneously.
Why You Should Learn RF Theory
Whether you’re studying radio theory to better understand your wireless network’s functionality, pass a certifying exam, or just make sense of RF, hopefully this collection of RF theory concepts has helped you. Believe it or not, the nature of electromagnetic waves, radio wave propagation and signal loss can be crucial knowledge for network administrators and technicians. This is just a small start to understanding radio theory. If you find yourself intrigued, get ready: electromagnetism is a fundamental force of the universe, and exploring it can be a fascinating experience.
delivered to your inbox.
By submitting this form you agree to receive marketing emails from CBT Nuggets and that you have read, understood and are able to consent to our privacy policy.